Monolithic Refractories Ceramic Lined Ceramic Ball Valve for Iron and Steel Industry
- Loading Port:
- Qingdao
- Payment Terms:
- TT OR LC
- Min Order Qty:
- 1000 pc
- Supply Capability:
- 3000 pc/month
OKorder Service Pledge
OKorder Financial Service
You Might Also Like
Application:
Applied in any media, expect HF (Hydrofluoric Acid) or glass solution, with max. temperature up to 500°C or max pressure 5.0 Mpa, such as high corrosion, high attrition and middle pressure.
Structural: 3-part flange type float ball valve Flange standards follow DIN/ANSI/API/JIS
l Excellent wear-proof property of ceramic enables this valve have high reliability and prolonged lifespan which is 2-4times as much as Titannium Alloy and Monel Metal valve.
l Elastic O ring fit between metal part and ceramic part makes the whole part high sealing capacity, unables the two parts blind, and avoid the ball broken.
l Valve steams are selected among 316/316L stainless steel, HC alloy, Monel Alloy, structural ceramic.
l Completely symmetric design ensures dual leak-proof and two-direction usage to prolong its lifespan to twice.
All parts that connect the medium are made of structural ceramics with extremely high chemical stability and hardness (HRC 90), which is only inferior to diamond. So the valve features exceedingly high wear-proof capability, corrosion resisting, enduring capability, good heat insulation, small thermal expansion.
The valve is unique in granule medium of high hardness, or erosive soft granule and is the only choice for such medium. They are wisely used in FDG System, Slag system and LNCFS in power plant, saline water and distilling processes in alkali works, paper pulp system in paper mill, and so on.
The ball is processed by advanced polishing equipment and technology that can ensure high circularity, good surface quality,.
The self-lubricating capability of ZrO2, ensure the good sealing performance between the ball and its seat. It is thoroughly free from the defects of easy leakage, big torque, non-resistance sealing surface comparing with metal sealing valve.
ZrO2 Composition Chart of MZ Ceramic Ball Valve
NO. | Physical Property | Parameter |
1 | Crystal | >85% cubic, the rest monoclinic |
2 | Lg. Loss | 0.8%-1.0% |
3 | Average | 0.4-0.7um |
4 | Apparent Density | >0.4g/cc |
5 | ZrO2 Purity | >99.95% |
6 | Tapped Density | >0.8g/cc |
- Q: How do monolithic refractories withstand the chemical attacks in copper smelting applications?
- Due to their unique properties and composition, monolithic refractories are capable of enduring chemical attacks in copper smelting applications. These refractories are specifically engineered to resist the harsh and corrosive environment found in copper smelting processes. To begin with, monolithic refractories are crafted from high-quality materials such as alumina, silica, and magnesia. These materials possess high melting points and chemical stability. Carefully selected, they are able to withstand the corrosive effects of copper smelting, including the presence of sulfur compounds and acidic gases. The refractory's composition also includes various additives and bonding agents that enhance its resistance to chemical attacks. In addition, monolithic refractories exhibit exceptional thermal shock resistance. This means they can withstand rapid temperature changes without cracking or spalling. In copper smelting applications, where extreme temperatures are involved, this refractory quality is crucial in preventing the formation of cracks and ensuring long-term performance. Furthermore, monolithic refractories possess a dense and compact structure. This structure serves as an effective barrier against the infiltration of molten copper and other corrosive substances. By preventing the penetration of chemical attacks, the refractory lining's durability and longevity are ensured. Moreover, monolithic refractories offer superior erosion resistance. This is particularly important in copper smelting applications, where high-velocity gases and flows of molten metal can cause erosion of the refractory lining. The refractory's erosion resistance prevents the degradation of the lining and maintains its structural integrity. In conclusion, monolithic refractories are specially designed to withstand the chemical attacks encountered in copper smelting applications. Through the use of high-quality materials, the incorporation of additives, and the possession of excellent thermal shock resistance, density, and erosion resistance, these refractories provide a reliable and durable lining that can endure the harsh conditions of copper smelting processes.
- Q: How do monolithic refractories contribute to the overall efficiency of iron and steel production?
- Monolithic refractories play a crucial role in enhancing the overall efficiency of iron and steel production. These refractories, which are made from a single material, provide exceptional thermal insulation, resistance to high temperatures, and excellent mechanical strength. By lining the furnaces, ladles, and other equipment used in the production process, monolithic refractories help in maintaining and regulating the required high temperatures for melting, refining, and shaping iron and steel. This insulation reduces heat loss, minimizes energy consumption, and ensures a more efficient and cost-effective production process. Additionally, the mechanical strength of monolithic refractories allows for better protection against wear and tear, resulting in increased equipment lifespan and reduced downtime for repairs and maintenance. Overall, the use of monolithic refractories significantly contributes to the efficiency, productivity, and sustainability of the iron and steel production industry.
- Q: What are the advantages of using low-cement castables in the iron and steel industry?
- There are several advantages of using low-cement castables in the iron and steel industry. Firstly, low-cement castables have a lower water content, which leads to better refractoriness and higher strength, enabling them to withstand high temperatures and thermal shocks experienced in iron and steel manufacturing processes. Secondly, low-cement castables exhibit excellent flowability and workability, allowing for easier installation and shaping. This results in reduced labor and time required for installation, leading to cost savings. Moreover, low-cement castables have a reduced porosity, which enhances their resistance to slag and metal penetration during the iron and steel production processes. This property improves the lining's durability and extends its service life, reducing maintenance and downtime. Additionally, low-cement castables offer superior mechanical properties, such as higher abrasion resistance and better thermal conductivity, making them ideal for various applications in the iron and steel industry, including ladles, tundishes, and blast furnaces. Overall, the advantages of using low-cement castables in the iron and steel industry include enhanced refractoriness, increased strength, improved workability, reduced porosity, and superior mechanical properties, ultimately resulting in improved efficiency, cost-effectiveness, and longer-lasting linings.
- Q: How do monolithic refractories resist chemical corrosion in iron and steel applications?
- Monolithic refractories resist chemical corrosion in iron and steel applications through their inherent properties and composition. They are designed to have high chemical stability and resistance to react with molten metals, slag, and other corrosive substances present in these applications. Additionally, monolithic refractories are usually formulated with specific additives and binders that enhance their resistance to chemical attack. This combination of properties and composition allows them to withstand the aggressive environment of iron and steel applications without significant degradation or corrosion.
- Q: How do monolithic refractories contribute to the overall efficiency of ladle slagging operations?
- Monolithic refractories contribute to the overall efficiency of ladle slagging operations by providing excellent thermal insulation, high resistance to thermal shock, and superior corrosion resistance. These properties allow for better heat retention, reduced heat loss, and extended refractory lifespan. As a result, ladle slagging operations can be carried out at higher temperatures with minimal refractory wear and tear, leading to increased productivity and cost savings. Additionally, monolithic refractories offer ease of installation and maintenance, ensuring quick turnaround times and minimizing downtime during ladle slagging operations.
- Q: What are the factors influencing the choice of monolithic refractories for different furnace types?
- There are several factors that influence the choice of monolithic refractories for different furnace types. Firstly, the operating temperature of the furnace is a crucial factor as different monolithic refractories have different temperature resistance levels. Secondly, the type of material being processed in the furnace is important as certain materials may require specific refractories to withstand their corrosive or abrasive nature. Thirdly, the furnace design and its heating method also play a role in determining the suitable refractory material. Additionally, the thermal conductivity, thermal shock resistance, and mechanical strength of the refractory are considered to ensure optimal performance and durability. Finally, cost, availability, and installation requirements are factors that can influence the choice of monolithic refractories for different furnace types.
- Q: How do monolithic refractories perform in reheating furnace roof applications?
- Monolithic refractories are highly effective in reheating furnace roof applications. These refractories are known for their excellent thermal shock resistance, which is crucial in the extreme temperature conditions inside a reheating furnace. They can withstand rapid temperature changes without cracking or spalling, ensuring the longevity and durability of the furnace roof. Additionally, monolithic refractories offer superior insulation properties, which help in maintaining the desired temperature inside the furnace. These refractories have low thermal conductivity, preventing heat loss and reducing energy consumption. This not only improves the energy efficiency of the furnace but also contributes to cost savings for the operators. Furthermore, monolithic refractories provide excellent resistance to chemical attacks from gases and molten metals present in the furnace environment. They are designed to withstand corrosive atmospheres and prevent the penetration of harmful substances, thus prolonging the life of the roof refractory. Moreover, monolithic refractories offer easy installation and repair options. Their ability to be cast or gunned in place allows for a seamless and precise application to the roof structure. This feature also enables quick and efficient repairs or maintenance, minimizing downtime and production losses. In conclusion, monolithic refractories are a reliable and efficient choice for reheating furnace roof applications. Their exceptional thermal shock resistance, insulation properties, chemical resistance, and ease of installation make them an ideal solution for maintaining the structural integrity and performance of the furnace roof.
- Q: What are some common applications of monolithic refractories in the iron and steel industry?
- Some common applications of monolithic refractories in the iron and steel industry include lining of ladles, tundishes, and converters, as well as repairs and maintenance of furnaces, kilns, and other high-temperature equipment. They are also used for the construction of runners, troughs, and spouts in continuous casting processes.
- Q: What are the typical compositions of monolithic refractories?
- Typically, monolithic refractories consist of a combination of aggregates, binders, and additives. Aggregates, such as alumina, magnesia, or silicon carbide, provide the main refractory properties and are chosen based on the desired characteristics of the refractory, such as resistance to high temperatures, thermal shock, or chemical attack. To bind the aggregates together and give the refractory its shape and strength, binders are used. Common binders include clay, calcium aluminate cement, or colloidal silica. The binder also impacts the overall performance of the refractory, affecting properties like thermal conductivity and chemical resistance. Additives are often included in the composition of monolithic refractories to improve specific properties or facilitate the processing. These additives can be plasticizers, which enhance workability and make installation easier, or antioxidants, which enhance the refractory's resistance to oxidation. Ultimately, the specific composition of a monolithic refractory can vary depending on the intended application and desired performance characteristics. Different combinations of aggregates, binders, and additives can be customized to meet specific temperature ranges, chemical environments, or mechanical stresses.
- Q: What are the benefits of using monolithic refractories in the iron and steel industry?
- There are several benefits of using monolithic refractories in the iron and steel industry. Firstly, monolithic refractories offer excellent thermal shock resistance, allowing them to withstand extreme temperature changes without cracking or spalling. This is crucial in the iron and steel industry, where materials are subjected to high temperatures during processes like melting, casting, and heat treatment. Secondly, monolithic refractories have superior corrosion resistance, making them highly durable against the corrosive effects of molten metals and slag. This is particularly important in the iron and steel industry, where materials come into contact with aggressive molten iron, steel, and various chemical compounds. Additionally, monolithic refractories provide excellent mechanical strength and abrasion resistance. They have the ability to withstand mechanical stress, vibrations, and impacts typically encountered in the iron and steel industry. This ensures longer refractory lifespan, reduces downtime for repairs or replacements, and improves overall operational efficiency. Moreover, monolithic refractories offer ease of installation and repair. Unlike traditional refractory bricks that require complex and time-consuming masonry work, monolithic refractories can be installed quickly and easily using simple methods like casting, gunning, or spraying. This saves time and labor costs during initial installation and subsequent maintenance or repairs. Furthermore, monolithic refractories provide flexibility in design and application. They can be tailored to specific shapes and sizes, allowing for customized linings in different parts of the iron and steel manufacturing process. This versatility enhances the overall efficiency and effectiveness of refractory linings, optimizing the production output and ensuring consistent quality of the finished iron and steel products. In conclusion, the benefits of using monolithic refractories in the iron and steel industry are numerous. They offer exceptional thermal shock resistance, corrosion resistance, mechanical strength, and abrasion resistance. They are easy to install and repair, and their flexibility allows for customized designs. Overall, monolithic refractories contribute to improved productivity, reduced downtime, and enhanced product quality in the iron and steel industry.
Send your message to us
Monolithic Refractories Ceramic Lined Ceramic Ball Valve for Iron and Steel Industry
- Loading Port:
- Qingdao
- Payment Terms:
- TT OR LC
- Min Order Qty:
- 1000 pc
- Supply Capability:
- 3000 pc/month
OKorder Service Pledge
OKorder Financial Service
Similar products
Hot products
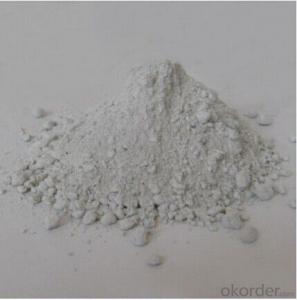
Monolithic Refractories for Iron and Steel Industry - Castable Refractory for Cement Kiln and Boiler
Hot Searches
Related keywords